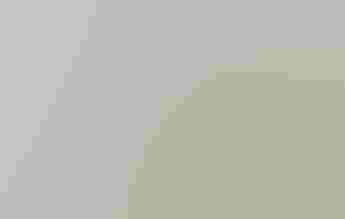
It was the day of the big flight test, and Obi the Pacific Parrotlet was ready. The small blue-green bird with an eight-inch wingspan had practiced its route—a short trip between two perches, three feet apart—for months. With help from assistants (with thumbs), Obi strapped on a pair of red safety goggles, which held tiny lenses cut from human-sized glasses to fit parrotlet-sized eyes. Once geared up, the captive-bred bird waited for its cue, and then took off through a fine mist of aerosol droplets, illuminated by lasers, to receive its reward of millet seeds on the other side.
Obi participated in three test flights led by robot-building engineers at Stanford University to better understand how birds fly. So far, the general theories behind avian flight hold that a wing’s curved shape generates lift to keep birds elevated; flapping generates thrust to propel them forward; and soaring allows birds to ride currents to save energy even while airborne.
But if you’re an engineer building avian-inspired robots, that basic understanding isn’t enough. Constructing a mechanical bird requires detailed knowledge of how wings move during flight, down to the smallest feather. As Lina Zeldovich reported in the summer 2016 issue of Audubon, that information still eludes experts:
“[T]here’s one thing the scientists agree on—how difficult it is to model a bird’s flight. For example, the birds’ wing movements are so complex that so far modern science hasn’t been able to precisely describe them using formulas and mathematical equations, according to David Lentink, a mechanical engineer who leads a bio-inspired engineering design lab at Stanford University in California. That’s the big reason it takes so many trials, errors, and crashes to build a bird.
Lentink is working to solve this problem. Using various mechanisms that he has developed to watch and measure the forces that birds generate with their wings as they fly, he hopes to understand how the animals achieve lift and propulsion, change their speed and direction, and adjust to wind gusts. He wants to fill in the gaps in understanding to help builders design better bots. Once the math is pinned down, he says, constructing small, safe, agile, and long-lasting flying machines would become much easier.”
Lentink is behind the new research starring Obi, which was published this week in the journal Bioinspiration & Biomimetics. In his efforts to develop a robot that can be maneuvered with wings, not propellers, he realized that he needed to better understand how a bird disrupts and displaces the air around it. By studying the airflow in the wake of a flapping wing, he could piece together how birds generate enough force to lift off and stay aloft.
Because airflow is typically invisible to the human eye, Lentink had to find a way to visualize it. To do that, he used lasers. Between Obi's perches, he created a laser sheet that illuminated microscopic aerosol droplets distributed in the air. As Obi flew through the sheet, wearing protective eyeware, a series of cameras recorded the movements of those droplets as they were moved aside by flapping wings and ensuing air currents. By reviewing the film, Lentink and his team could see precisely how the parrotlet propelled itself forward. They found that small whirlpool-like vortices of air formed behind its wings, similar to those generated by swimmers moving through water.
Lentink then compared Obi’s flight pattern to existing mathematical models used by engineers who were studying bird aeronautics and building small robots. Those models showed smooth vortices, like donut rings, that form and persist behind each wing beat. But none of the models were correct; the vortices generated by Obi’s flight were more complex and violent over the course of multiple wing beats.
This new discovery will send engineers back to the laboratory as they strive to accurately model the mechanical forces behind avian flight and lift force. Ultimately, that should produce better robots. And for that, they can thank Obi, the brave begoggled parrotlet.